Novel and Sustainable Binder Systems
High volume limestone in cementitious systems: physico-chemical effects and properties
The environmental impact, particularly the significant emission of greenhouse gases associated with the manufacture of ordinary portland cement (OPC) has catalyzed studies on the use of several environmentally benign alternate materials as partial OPC replacements. Fine limestone powder is one such material that has shown to be a viable partial OPC replacement material. A clear understanding of the effects of limestone replacement in combination with fly ash or metakaolin on the fresh and hardened properties has facilitated new strategies to proportion binder formulations which show comparable or superior properties to traditional OPC formulations.
Limestone can be interground with OPC clinker in the cement manufacturing process or blended with the cement during the concrete batching process. Improved particle packing, fine limestone acting as nucleation sites for reaction product formation, and consequently better properties are obtained. Studies on the use of fine limestone as a partial cement replacement material, and its synergy with other aluminate-bearing materials such as fly ash, metakaolin, and slag have been investigated in great detail. The hydration process in such
systems, mechanical property development, and rheological characteristics are elucidated in many of our publications. Through the use of mathematical models and accelerated transport experiments, we have also established the beneficial effects of limestone containing ternary blends on concrete durability.



Iron based binders – Waste Utilization and carbon dioxide sequestration




Methods to reduce the amount of greenhouse gases (GHG) such as CO2 in the atmosphere is an active research area.
Mineral route of carbonation, where mined mineral rocks are used as the feedstock through which CO2 is passed is one of the promising routes to reduce the concentration of CO2 in the atmosphere. We have forwarded, for the first time, the possibility of carbonating waste metallic iron powder to develop sustainable binder systems for concrete. The fundamental premise of this work is that metallic iron will react with aqueous CO2 under controlled conditions to form complex iron carbonates which have binding capabilities.
We have studied the effect of source materials, additional components including the source of silica and alumina, nucleation agents, iron dissolution agents and curing conditions on the microstructure and mechanical properties of this unique binder system. The reaction kinetics and product formation have been determined and modeled. The material pore structure is investigated primarily through mercury intrusion porosimetry whereas electron microscopy is used for microstructural characterization. The pore structure features are used in predictive models for gas and moisture transport (water vapor diffusivity and moisture permeability) through the porous medium which dictates its long-term durability when used in structural applications. Comparisons of the pore structure with those of a Portland cement paste are also provided. The morphology of the reaction products in the iron-based binder, its elemental composition and the distribution of constituent elements in the microstructure are also reported. The novel binder system provides yet-unheard-of synergistic benefits through: (i) consumption and trapping of CO2 from a GHG emitting industrial operation and subsequent carbonate formation, and (ii) providing a means to reduce the overall Ordinary Portland Cement (OPC) production (which is a significant emitter of GHG) through the use of carbonated metallic iron powder as the binder material for concrete.
Alkali Activation of alumino-silicates
Several strategies are being recommended and adopted towards reducing the production of ordinary Portland cement (OPC) which is proven to be a large contributor of greenhouse gas emission. Among these methods, one of the most studied one is the development of binder systems using alkaline activation of aluminosilicate materials such as fly ash (coal combustion by-product), or ground granulated blast furnace slag. This methodology is extremely beneficial in concrete because the process of forming a value-added material by utilizing large volumes of an industrial waste/byproduct alleviates concerns related to its disposal and results in a lower ecological foot-print for the concrete thus produced.
Our work on alkali activated fly ash (geopolymers) and slag systems have spanned studies on reaction mechanisms and product formation, microstructural studies, and mechanical and durability properties. We have explored the influence of the alkali cation type on the reaction kinetics, compressive strength, and reaction product structure in alkali activated slag and fly ash systems through advanced experimental techniques such as NMR spectroscopy. We have employed advanced microstructural analysis tools such as synchrotron x-ray tomography to understand the microstructure and implement predictive models. Extensive studies on transport performance of alkali activated systems using accelerated transport methods and electrical impedance-based methods have been carried out. Our focus is also on the use of less caustic and neutral activator materials for these systems as well as energy-efficient curing methods.



Rheological response of cementitious binders
Rheological studies of concentrated suspensions of solid particles in a continuous liquid medium are commonly used to assess the characteristics of materials in industries ranging from food to pharmaceuticals to construction materials. The flow behavior of these concentrated suspensions is influenced by surface contacts between solid particles and interparticle forces such as van Der Waals and steric forces. We have developed methods to extract yield stress of cementitious suspensions without approximating models and elucidated the rheological response of several sustainable binders.
Rheological studies of cementitious suspensions provide an understanding of how these materials behave in the fresh state and serve to monitor structure development that dictates the development of the mechanical properties. The influence of particle packing, particle contacts, surface areas, water film thickness, and solid loading on the yield stress and plastic viscosity of suspensions are evaluated in detail. Fundamental rheological studies are also used to infer the surface interactions between particles in multi-particle suspensions, either in water or a concentrated alkali solution (such as in the case of alkali activated systems). Relationships between rheological parameters and solid loading are arrived at to obtain better material designs for desirable fresh properties.
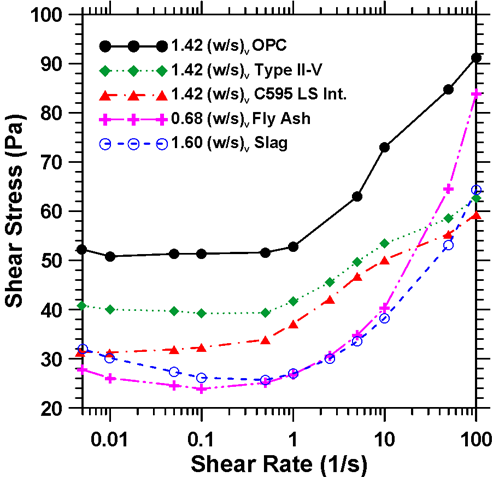
