Research
Our group seeks to dramatically improve the size, efficiency, operating range, and performance of power electronics and leverage these improvements to move energy systems forward.
In pursuing this goal, we target three overarching research thrusts: innovative converter designs and architectures which mitigate the passive component bottlenecks in power electronics, improved techniques and approaches for power magnetic components, and exploring new/emerging applications that can be enabled/supported by power electronics. This page summarizes some of the ongoing work in our laboratory. Please feel free to contact us if you would like to discuss further.
We gratefully acknowledge our research partners and the organizations that support our research:
These research thrusts are displayed below in order of component-level, then converter-level, then system-level work performed by our group.
Automated Core Loss Characterization
Magnetic components present a key bottleneck towards miniaturizing power electronics. Understanding the loss of a magnetic material is foundational to maximally leveraging their capability and mitigating this bottleneck. A difficulty is that these losses are generally non-linear and must be assessed under real-world large-signal excitations. Today, this data is very limited, typically reported using empirical power-law fits on a select amount of measured data (the Steinmetz equation). We have developed, and continue to improve, in-house core loss testers that enable automated collection of core loss data under varying waveshapes, flux densities, frequencies, and temperatures (25-100C), including automated core loss testing at 1-20MHz. These testbeds enable identification of the highest performing magnetic materials for miniaturization.
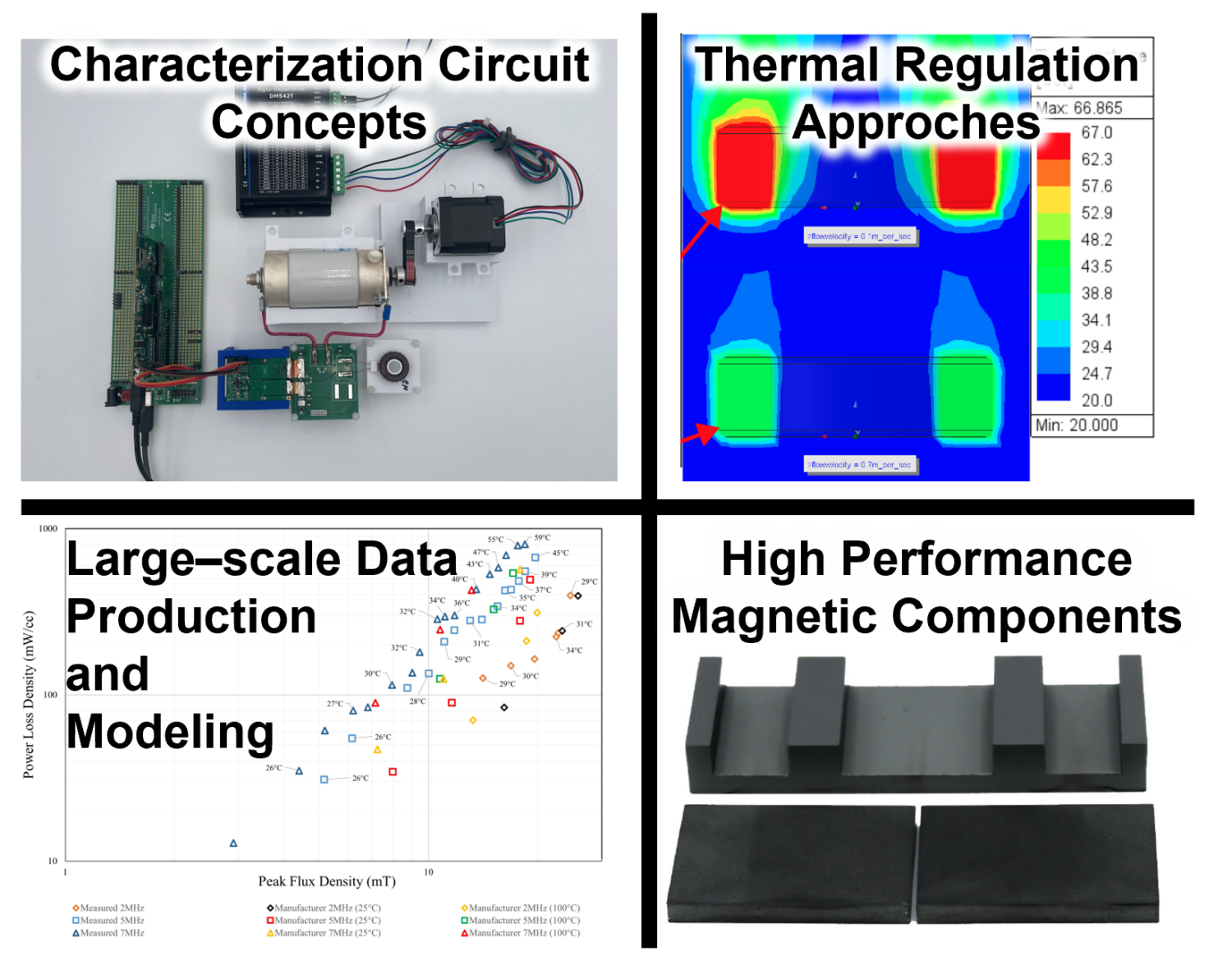
High Performance, High Frequency Planar Magnetics
Planar magnetic designs are especially promising for miniaturization,
offering strong advantages in terms of manufacturability, repeatability, thermal performance and cost compared to wire-wound alternatives. However, they present challenges such as poor window fill factor, the potential for high inter- and intra-winding capacitance, and the difficulty of achieving highly efficient
terminations, especially in highly interleaved designs. These challenges are aggravated at the higher operating frequencies where many high performance magnetic materials are optimally operated. We work to better understand these challenges and limitations, and to develop designs which are optimized around them. We are also exploring new domains for this technology, such as for low-cost medium voltage isolation in utility-scale solar photovoltaic power plants.
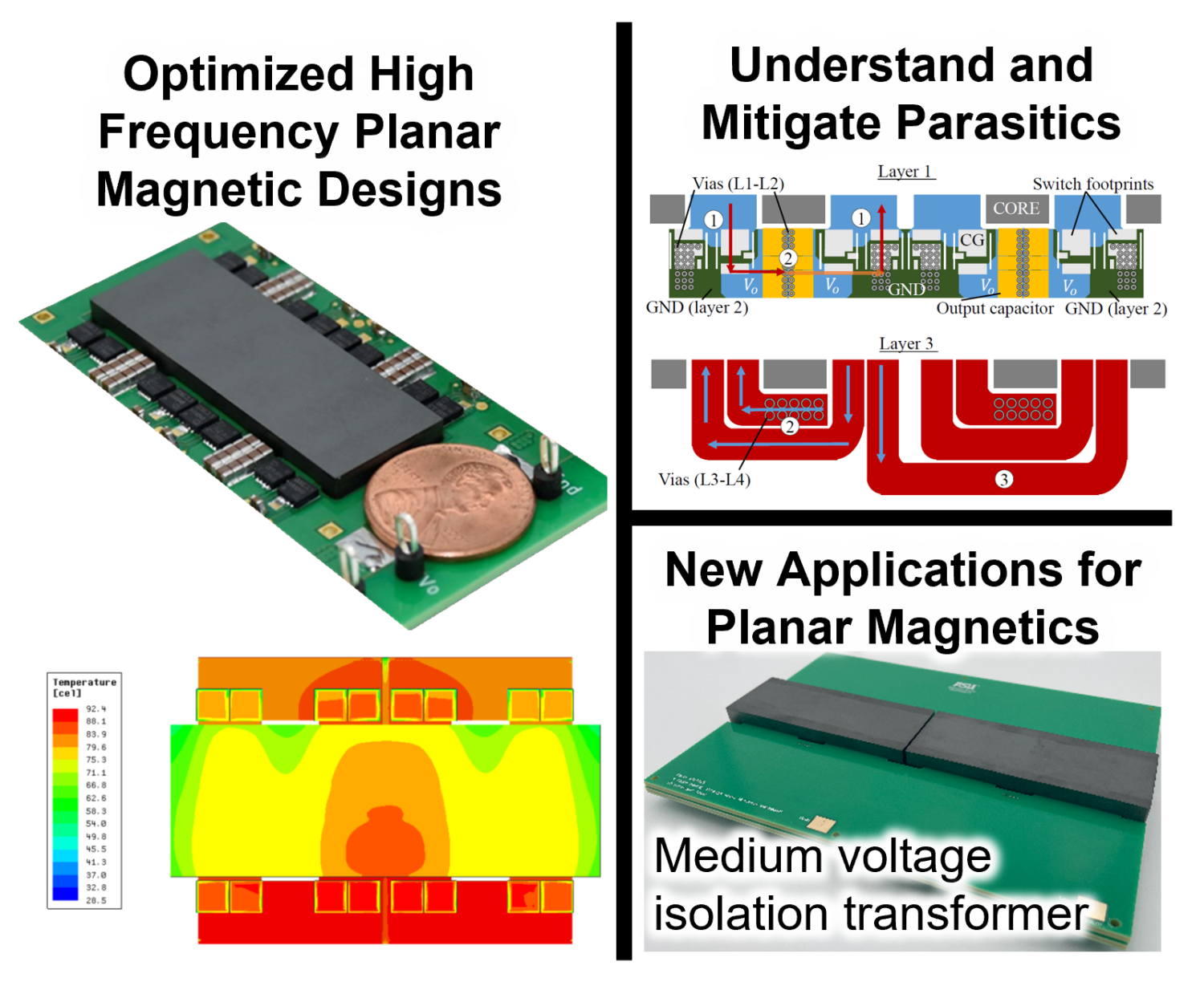
Coupled Electronic and Magnetic Systems
Our research group leverages a special magnetic component architecture which we call a “Coupled Electronic and Magnetic System” (CEMS). In a CEMS, electronics define the conductive loops around one or more magnetic cores such that those electronics become fundamental to defining the magnetic properties of the component and vice-versa. This can enable new and useful functionality compared to a conventional connection between those electronics and magnetics. An example CEMS is the Variable Inverter/Rectifier Transformer (VIRT), illustrated here. This structure enables the two full-bridges formed by Q1-Q4 and Q5-Q8 to be dc coupled but ac decoupled (taking advantage of the transformer core to also provide this ac decoupling). This allows a voltage greater than the output voltage to be applied to the transformer’s center post, and results in this structure behaving like a transformer with “fractional turns” (e.g., 4:1 step down with only 2 primary turns), highly advantageous for high step-down, high output current applications. Our group explores this and other CEMSs to maximize the capability of magnetics.

Miniaturized Power Converters
We combine our expertise in miniaturized high frequency magnetics, planar magnetics, and CEMSs with emerging wide-bandgap semiconductor devices and resonant/soft-switching circuit topologies to develop miniaturized and advanced power converters.
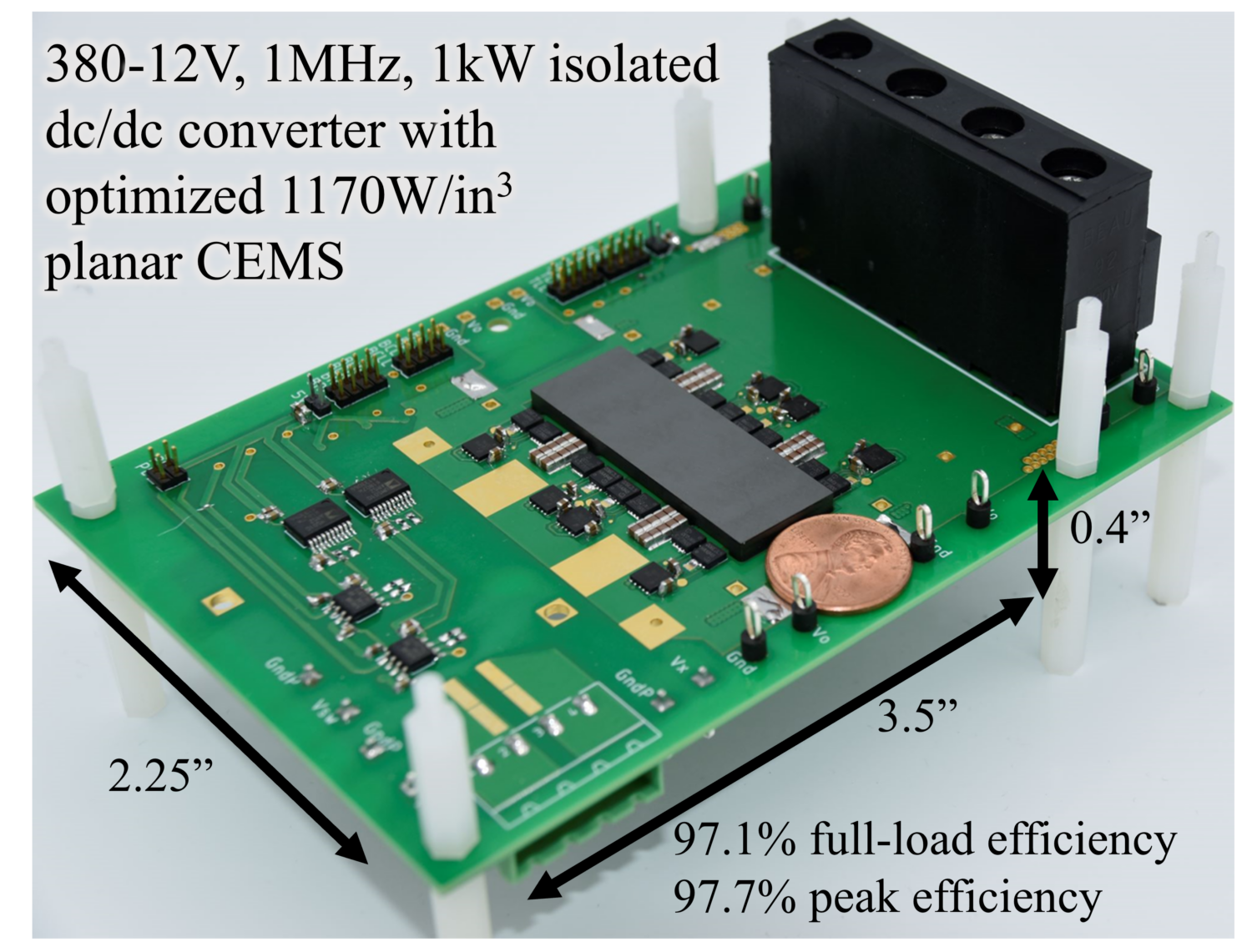
RF Power Conversion and Applications
Our laboratory can support power electronics research in the low ISM bands (6.78, 13.56, 27.12 MHz). These frequencies are relevant for industrial RF power generation (e.g., plasma generation systems for semiconductor fabrication) and wireless power transfer. An example relevant project is a 13.56MHz variable reactor platform we developed to mimic the time-varying reactance of a plasma load, pictured here. This can be combined with a time-varying resistance platform to implement a “plasma dummy load” that shortens the development and debug time for evaluating new RF plasma generation concepts and systems.

Global HyPT Center
The Global Hydrogen Production Technologies (HyPT) Center establishes an international partnership of six countries – US, Australia, Canada, UK, Egypt, and Germany – to formulate a pathway to low-cost large-scale net-zero hydrogen production, with funding from US, Australia, Canada, and UK. Researchers with diverse expertise from many institutions in different countries interweave under the Center to work synergistically with the common goal of achieving US$1/kg and gigatons/year net-zero hydrogen production. Our group is exploring power electronic architectures towards this goal.
